Method of Oligonucleotide PurificationMethod of Oligonucleotide purification depends on three key factors:
The first factor is the length of an oligonucleotide. The longer an oligonucleotide sequence is, the more failing sequences present in the crude mixture. Regardless of coupling efficiency, long oligonucleotides of greater than 50 bases are highly recommended to undergo purification. The second factor is application. For example, some oligonucleotides require characterization by ion exchange chromatography to achieve extremely high purity greater than 98%. This is important for cell viability. The preferred method of purification is anion exchange HPLC due to this ability to produce high quality oligos in the cell friendly Na+ salt form. The third factor is modifications. Sometimes a labeled or dual labeled oligo is required for molecular beacon, FRET, biotin-streptavidin, or bio conjugation applications. In these special cases, the hydrophobicity of the modifying group at either the 5’ or 3’ end can be utilized to produce high quality oligos through C18 reverse phase HPLC. The most common purification techniques used at Biosynthesis are:
Each purification technique has its own strengths and weaknesses. However, if done properly each purification method can produce high quality oligonucleotides. PAGE: Polyacrylamide gel electrophoresis is the oldest technique in oligonucleotide purification. Generally, a high percentage acrylamide gel is used to separate the full length products from all failed sequences based on their charge and ability to “run” through the gel matrix. PAGE can be used to separate oligos with lengths greater than 15 bases all the way up to 400 base pairs yielding purities greater than 90%. The advantage of PAGE is that it is the only purification technique that can resolve unmodified oligos of lengths greater than 80 bases. Biosynthesis always recommends long oligonucleotides greater than 80 bases to be purified by PAGE. However, there is one drawback. PAGE purified products will yield a lower final purified product compared to HPLC because it is impossible to recover every bit of full length product from the gel slice. However, the loss in final product is an acceptable tradeoff if high quality, unmodified long oligonucleotides are needed. Shown below in Figure 1 is an analytical electropharrogram of a 218 base oligonucleotide made and purified by Biosynthesis. ![]() Fig 1.Analytical electropharrogram of a PAGE purified unmodified 269 base oligonucleotide. The band clearly shows that all truncated sequences were removed. RP HPLC: Reverse phase is generally the “go-to” standard for HPLC purification of oligonucleotides due its ability for quick mass spec and analytical HPLC quality control of fractions. General knowledge of how RP HPLC works is important to understanding the purification efficiency of RP HPLC. Many find it interesting that RP HPLC actually separates failed oligos from full length products by charge. How is it that a technique based on hydrophobicity of a compound can separate a polar molecule? In reality, RP HPLC of oligonucleotides is actually “ion-pairing chromatography”. This is a technique in which a long-chained alkyl amine is added in low concentration to the mobile phase in order to achieve enhanced resolution. Below is a schematic (scheme. 1) of how an oligonucleotide interacts with in ion-pairing reagent which in turn interacts with the C18 stationary phase of the column. ![]() Scheme 1.Interaction of an oligonucleotide with an ion-pairing reagent at the surface of a C18 support. This “indirect” interaction is the reason why RP HPLC can only resolveoligos from 8 to 40 bases with greater than 90% purity. However, if greater purity is required, the hydrophobic DMT group of the oligo can be left on the last 5’ base during synthesis, producing greater than 95% pure oligonucleotides. The advantage of both forms of HPLC (RP and AEX) over PAGE is that the yield is typically much higher relative to the starting scale. A second advantage to reverse phase chromatography is its ability to resolve modified oligos from unmodified oligos. Typically, modifications at the 5’ or 3’ end of an oligonucleotide such as fluorophores, biotin, amino,sulfohydryl, etc. are hydrophobic enough to interact directly with the stationary phase of the C18 column. This leads to highly purified custom modified oligos that can be greater than 95% pure. The two disadvantages to RP HPLC are(unmodified) oligos greater than 40 bases and less than 8 bases cannot be resolved. It is important to point out that RP HPLC purified oligonucleotides are left in the ion-pairing reagent salt form. Normally, this is the triethylammonium amine (TEAA) salt. If the oligonucleotide is to be used in living systems such cells or animals, TEAA is very toxic. A simple sodium salt exchanged can be performed to remove any TEAA that would cause harm to cells. Shown below in fig. 2, fig. 3, and fig. 4 is a preparatory chromatogram,mass spectra, andanalytical report, respectively, of a 9mer 3’ Atto 655 modified oligonucleotide that was made and purified by Biosynthesis. ![]() Fig 2.Semi-preparatory RP HPLC chromatogram of a crude 9mer 3’ Atto 655 modified oligonucleotide. The peak at 9.85 minutes represents the unmodified oligonucleotide. The main peak represents the highly pure Atto 655 modified product. ![]() Fig 3.Mass Spectra of main peak collected from preparatory RP HPLC of the 9mer 3’Atto 655 modified oligonucleotide. The calculated molecular weight is 3433.55. The observed molecular weight is 3434.46. ![]() Fig 4.Analytical HPLC report of 9mer 3’ Atto 655 modified oligonucleotide of main peak from preparatory RP HPLC. Fluorophores such as Atto 655 contain enough hydrophobicity to allow for excellent reverse phase separation yielding high purity oligonucleotides. AEX HPLC: Anion-exchange chromatography is an established technology with a long history. AEX HPLC is the best form of purification for unmodified oligonucleotides up to 80 bases. The difference between AEX HPLC purification and RP HPLC purification is the way oligonucleotides interact with the stationary phase. In RP HPLC, the interaction was indirect. However, in AEX HPLC the negatively charged phosphate backbone of the oligonucleotide can directly interact with the positively charged stationary phase of the anion exchange column. This results in higher resolution. There are 3 main advantages to AEX HPLC. The first advantage is that oligomers up to 50 bases can be greater than 95% pure. From 50 to 80 bases, 95% or greater purity can also be achieved, but at lower yields. The second advantage is that AEX HPLC is the only type of purification technique that can successfully separate phosphothiolate, LNA, and BNA modified oligonucleotides. Generally, Biosynthesis recommends AEX HPLC when these modifications are present. However, if a hydrophobic modifying group is present, RP HPLC can also be used. The last advantage is that oligos that elute from an AEX HPLC system are in the Na salt form ready for use in living cells. The only minor setback of AEX HPLC is its slightly higher cost compared to RP HPLC and its lower resolving capability of 3’ or 5’ modifiedoligos. In such cases many customers chose DUAL HPLC purification with both RP and AEX HPLC to achieve 98% or greater purity. Shown below in Fig. 5, Fig. 6, and Fig. 7 is a preparatory chromatogram, mass spectra, and analytical report respectively of a 28mer oligonucleotide purified by Biosynthesis. ![]() Fig 5.Semi-preparatory AEX HPLC chromatogram of a crude 28mer oligonucleotide. Failure sequences are represented as peaks before the main peak. The small peaks after the main peak are sequences that are not fully de-protected. ![]() Fig 6.Mass Spectra of main peak collected from preparatory AEX HPLC of the 28mer unmodified oligonucleotide. The calculated molecular weight is 8531.58. The observed molecular weight is 8532.17. ![]() Fig. 7Analytical AEX HPLC report of the unmodified 28mer oligonucleotide from the main peak of the preparatory AEX HPLC. Purities exceeding 95% in this case 97.8% are commonly obtained when using AEX HPLC to purify oligonucleotides. Whatever purification methods our customers decide to choose, Biosynthesis is committed to synthesizing the purest oligonucleotides. |
Method of Oligonucleotide Purification
Ac-SDKP peptide (also known as seraspenide or goralatide)
The synthetic tetrapeptide Ac-SDKP, also called seraspenide or goralatide, has the chemical structure 1-(N2-(N-(N-acetyl-L-seryl)-L-alpha-aspartyl)-L-lysyl)-L-proline, the molecular formula C20H33N5O9, and a molecular weight of 487.50412 dalton. This tetrapeptide is involved in angiogenesis and the regulation of the immune response by blocking the proliferation of lymphocytes. It is generated by the cleavage of thymosine beta4 and inhibits the proliferation of hematopoietic stem cells and the proliferation and secretion of fibroblasts in the myocardium and the glomeruli. Lymphocytes are a type of white blood cells in the vertebrate immune system and are part of the adaptive immune system. The seraspenide peptide inhibits cell cycle entry of normal hematopoietic stem cells. Ac-SDKP protects hemopoiesis, the blood cell formation from established blood cell precursors, against damage caused by cytarabine, cyclophosphamide and carboplatin in mice. Hematopoietic stem cells give rise to all other blood cells. The renin–angiotensin system, a hormonal system that regulates blood pressure and water or fluid balance in mammals, is central to regulation of blood pressure and electrolyte homeostasis. N-Ac-Ser-Asp-Lys-Pro is an inhibitor of primitive hematopoietic cells and has been reported to block the proliferation of clonogenic cells freshly isolated from normal marrow and cord blood. Ac-SDKP can cause a transient decrease in nonadherent cell and progenitor output when added repeatedly to normal long-term marrow cultures. Ac-SDKP is detectable in experiments with marrow from patients with chronic myeloid leukemia. Clonogenic cells are cells that have the potential to proliferate and to give rise to a colony of cells. Only some daughter cells from each generation retain the potential to proliferate. The angiotensin-converting enzyme, a key protease, has a range of substrates, including N-acetyl-Ser-Asp-Lys-Pro (Ac-SDKP). The peptide Ac-SDKP is cleared almost exclusively by the angiotensin-converting enzyme. N-Acetyl-Ser-Asp-Lys-Pro is a negative regulator of haematopoietic stem cell differentiation and a potent antifibrotic agent. AZT (3'-azido-3'-deoxythymidine), the main antiviral drug used in AIDS treatment, is toxic to hematopoietic progenitor cells and is known to induce anemia, a decrease in number of red blood cells, and neutropenia, the abnormally low number of neutrophils or white blood cells. The tetrapeptide AcSerAspLysPro (AcSDKP, Seraspenide) has been shown to increase the survival of mice subjected to high doses of chemotherapy and to reversibly block the cycling of human granulocyte-macrophage colony forming unit and burst forming unit erythroid progenitors. Furthermore, it has been suggested that AcSDKP may be an efficient factor in preserving progenitors against AZT-induced hematopoietic toxicity. Research indicates that the peptide could protect hematopoietic stem cells during anti-neoplastic treatments leaving cancerous cells unprotected. The tetrapeptide opposes the effects of TGFbeta and could prevent fibrosis after myocardial infarcts and glomeruli fibrosis during the natural course of diabetic nephropathy. Reference Ross G Douglas, Mario R Ehlers and Edward D Sturrock; Evolving Concepts of the Renin–Angiotensin System Antifibrotic peptide N-acetyl-Ser-Asp-Lys-Pro (Ac-SDKP): Opportunities for angiotensin-converting enzyme inhibitor design. In Frontiers in Research Review: Clinical and Experimental Pharmacology and Physiology (2013), 40, 535–541. |
Size Exclusion Chromatography
sabi test
Neuropeptides for Neuroscience Research
Neuropeptides for Neuroscience ResearchOne of the greatest challenges in neuroscience research is to understand how biologically active molecules such as small molecules and peptides function at different levels, starting at the atomic level to the ensembles of neuronal networks. Advances made in chemical and synthetic biology over the last few decades have given researchers new insights into bioactive molecular actions. One important key feature that has emerged in recent years is the observation that adult mammalian brains exhibit much more plasticity and regenerative capacity than previously thought. During neurogenesis, the process by which neurons are generated from neural stem and progenitor cells, adult brains are able to generate functionally integrated new neurons. Progenitor cells are ancestor cells that can differentiate into specific cell types. Neuroscientist are just now beginning to study the basic properties of adult neural stem cells and the molecular, cellular and circuitry mechanisms that regulate the sequential adult neurogenesis process in vivo. These studies include the new field called “neuroepigenetics” that investigates the function of novel active DNA modifications and their physiological role in the nervous system. The discovery of neuropeptides in the mammalian nervous system started a revolution in traditional physiology. Groundbreaking research in physiology, endocrinology, and biochemistry during the last century has let to this discovery. The three notions governing peptide research in the early years of peptide based neuroscience are: (1) peptide hormones are chemical signals in the endocrine system; (2) neurosecretion of peptides is a general principle in the nervous system; and (3) the nervous system is responsive to peptide signals. These ideas have contributed to how neuropeptides are defined today: “Neuropeptides are small substances similar to proteins produced and released by neurons through the regulated secretory route that act on neural substrates.” The beginnings of neuropeptide research can be traced back to the histories of physiology, pharmacology and biochemistry. Originally the term “neuropeptide” indicated a small protein molecule present in neurons. However, in the late 1970s and the 1980s of the last century, many neuropeptides were localized by immunocyto-chemistry to discrete cell populations of the central and peripheral nervous system leading to the concept of “chemical neuroanatomy” as part of neurobiology. However, recently the field of neuropeptide research or biology has dramatically expanded and today the research goals involve the development of pharmacologically active compounds that are capable of crossing the blood–brain barrier to exert their biological function(s) in vivo and in the construction of genetic vectors useful for gene therapy. How are neuropeptides defined today?The term ‘neuropeptides’ was first introduced by D. de Wied in 1971 to describe fragments of peptide hormones lacking the activity of the intact hormone molecule but that were able to produce behavioral changes. Subsequently peptide hormones, their fragments, endogenous opioid or morphine-like peptides as well as a large number of other biogenic peptides became classified as neuropeptides. All these peptides are classified based on a number of common features including their origin, biosynthesis, secretion, metabolism, and effectiveness. Neuropeptides are found in the nervous tissue, in peptide-secreting cells present in various organs such as the gut, placenta, heart, lungs, the immune system and other tissues, and are active at extremely low concentrations. The more modern definition of a neuropeptide is as follows: “A neuropeptide is a peptide found in the nervous system which has biological effects when injected.” Neuropeptides have now been found to be the most diverse class of signaling molecules in the brain that participate in many physiological functions. Almost 70 genes have been identified in the mammalian genome to encode for neuropeptide precursors and a multitude of bioactive neuropeptides. Unfortunately the boundary between a neuropeptide and a neuroprotein can be blurry. This is exemplified in the findings that there are several subfamilies of peptides and small proteins which display most of the hallmarks of neuropeptides. For example among cytokines, peptide hormones, and growth factors there are several subfamilies of peptides that perform similar functions as neuropeptides such as neural chemokines, cerebellins, neurexophilins, and granins. The "cytokine" family alone includes a large and diverse family of regulators produced throughout the body by cells of diverse embryological origin. The use of in situ hybridization and immunocytochemistry allowed scientists to unambiguously demonstrate for many neuropeptides that the transcript and peptide products are produced by neurons. For a few neuropeptides the biosynthesis of the peptides could be demonstrated by the incorporation of radioactive amino acids using pulse-chase experiments. Why are neuropeptides important?The recent Annual Neuroscience Meeting held in San Diego from November 9 to 13 demonstrated the importance of all classical neuropeptides as well as of new putative neuropeptides for brain research. The meeting was attended by more than 30,000 neuroscientists and students and the forest of presented posters was so huge that one was only able to visit a selection of them during the meeting hours. One particular peptide that stood out of the crowd is the NAP peptide. This peptide offers new hope to find a cure to prevent the onset of Alzheimers disease or any similar disease, and, hopefully, a few other neuro-degenerative diseases that involve protein unfolding or misfolding events in the mammalian and human brain. Neuropeptides can be neuroprotective!“NAP peptide (Asn-Ala-Pro-Val-Ser-Ile-Pro-Gln) is a neuroprotective peptide that shows cognitive protection in patients with amnestic mild cognitive impairment, a precursor to Alzheimer's disease. NAP exhibits potent neuroprotective properties in several in vivo and cellular models of neural injury. While it has been found in many studies to affect microtubule assembly and/or stability in neuronal and glial cells at fM concentrations, it has remained unclear whether it acts directly or indirectly on tubulin or microtubules. We analyzed the effects of NAP (1 fM-1 µM) on the assembly of reconstituted bovine brain microtubules in vitro and found that it did not significantly (p < 0.05) alter polymerization of either purified tubulin or of a mixture of tubulin and unfractionated microtubule-associated proteins. NAP also had no significant effect (p < 0.05) on the growing and shortening dynamics of steady-state microtubules at their plus ends, nor did it alter the polymerization or dynamics of microtubules assembled in the presence of 3-repeat or 4-repeat tau. Thus, the neuroprotective activity of NAP does not appear to involve a direct action on the polymerization or dynamics of purified tubulin or microtubules.” [Source: Mythili Yenjerla, Nichole E LaPointe, Manu Lopus, Corey Cox, Mary Ann Jordan, Stuart C Feinstein, and Leslie Wilson; The Neuroprotective Peptide NAP Does Not Directly Affect Polymerization or Dynamics of Reconstituted Neural Microtubules. J Alzheimers Dis. 2010 January 1; 19(4): 1377–1386. doi: 10.3233/JAD-2010-1335. PMCID: PMC2844470, NIHMSID: NIHMS179597.] Gene expression, biosynthesis and regulated release in neuronsNeurons use neuropeptides for signaling to other cells. Expression of the genes coding for neuropeptides and their biosynthesis occur in neurons. This has been shown using in situ hybridization and immunocytochemistry. Usually only peptides produced in the nervous system by neurons are called neuropeptides. However, astrocytes and glial cells apparently can also have a regulated secretory pathway and therefore, putative neuropeptides may be recognized in peptide families expressed by glial cells. Recent research suggest that many neurotransmitters, neuropeptides and calcium binding proteins appear early during development of the cerebellum, have specific temporal and spatial expression patterns, may have functions other than those found in the mature neural systems, and may be able to interact with each other during early development. The basis for regulation of chemical communication is controlled secretion of the signal molecules involved. Neurons expressing classical neuropeptides use the regulated secretory pathway. In this pathway biosynthesized peptides are stored in large dense-cored vesicles and released in a controlled manner upon a stimulus. A signal peptide sequence is needed for entry of the newly synthesized gene product into the lumen of the endoplasmic reticulum (ER) as part of the secretion routes. Usually the signal peptide is short, containing 20 to 25 amino acids at the N-terminal end of the precursor, called the prepro-peptide. This signal peptide is removed from the nascent precursor during protein synthesis when it passes through the ER membrane, leaving the pro-neuropeptide in the ER-Golgi for further sorting into the regulated secretory pathway. Next, neuropeptide precursors are sorted in the trans-Golgi network into the vesicles of the secretory pathway. The resulting protein content is acidified and condensed in the vesicles, and activated proteolytic enzymes process the precursor protein into neuropeptides. Pairs of the basic amino acids lysine (Lys, K) and arginine (Arg, R), or, sometimes, a single Arg in the appropriate structural environment of the precursor, serve as recognition sites and substrates of prohormone convertases (PCs). Next, differential cleavage of the neuropeptide precursor by the proteases leads to the generation of specific peptides and cell-specific different sets of peptides from the same precursor. Peptides generated in this way can be subject to further modification by peptidyl-aminotransferase (PAM). This enzyme uses a C-terminal glycine (Gly, G) as amide donor for the preceding amino acid which results in a peptide with an amidated C-terminus. This amide group has been found to occur in many neuropeptides and is often essential for its biological activity. Furthermore, many neuroppetides have been found to contain post-translational modifications including N- and O-glycosylation, phosphorylation, sulfation, and acetylation. These are found on stored and secreted peptides and contribute to their biological properties. The resulting neuropeptides are stored in mature granules or dense-cored vesicles where they await release upon a stimulus. Neuropeptides can function as hormonesSome neuropeptides can also function as hormones. A hormone is defined as a substance secreted by one organ and acting on another after transport by the bloodstream. This allows cells at different locations of an organism to communicate in an endocrine fashion. Since many peptide hormones are also synthesized by neurons these peptides therefore belong to the class of neuropeptides. However, many classical neuropeptides are also synthesized by endocrine glands and function peripherally as hormones which can make the classification of these peptides somewhat confusing. By definition hormones are excluded as being neuropeptides if they are not synthesized by neurons, even if they signal to the brain. Leptin and insulin are examples. In addition, sometimes the relationships between the nervous system and peripheral endocrine glands are complicated by alternative processing and splicing. Hormones are defined as being chemical substances formed or synthesized in one or part of an organ in the body and are carried in the blood to another organ or part. Depending on the specificity or effect of the hormone the targeted organ may be altered in its functional activity or even in its structure by the effect of the hormone. As new peptides are discovered in various tissues that can function as neuropeptides the current classification schemes may need to be expanded. |
Peptide Design for Immunization using Multiple Antigen Peptides
How to design peptides useful for immunization using multiple antigen peptides (MAPs)The design of peptides to generate antibodies against protein epitopes is still considered an art. However, experienced researchers can use an educated approach for the design of immune response inducing peptide sequences for example by determining the amino acid sequence regions exposed to the surface of a protein. One approach is to review the folding of proteins and to search for β-turn loops where the amino acid residues at the turns are pointed to the surface of the proteins. This approach works well for proteins for which the 3D structures are available in a public data base such as the Pubmed Structure database [http://www.ncbi.nlm.nih.gov/structure/] or the RCSB PDB database [http://www.rcsb.org/pdb/home/home.do]. Another approach is to screen the protein sequences for sequence regions that have a high probability to induce immunization using bioinformatic methods. The following web link can help to achieve this: http://www.proteinlounge.com/biosyn/pepfinder_home_in.aspThe multiple antigen peptides (MAP) system represents another approach to anti-peptide antibody elicitation. It has been reported that MAPs can be used for the production of high-titer anti-peptide antibodies as well as synthetic peptide vaccines. The system was first described by Dr. James Tam. A MAP contains a small immunologically inert core molecule of radially branched lysines dendrites onto which a number of peptide antigens are anchored. The result is a large macromolecule which has a high molar ratio of peptide antigen to core molecules and does not require further conjugation to a carrier protein. The α- and ε-amino functional groups of lysine residues are used to form a dendrimeric type core to which multiple peptide chains are attached. Depending on the number of lysines used to synthesize the core structure different numbers of peptide branches can be synthetically attached.
|
Propionylated and Acetylated Peptides
References
Poonam Bheda, Jennifer T. Wang, Jorge C. Escalante-Semerena, and Cynthia Wolberger; Structure of Sir2Tm bound to a propionylated peptide. PROTEIN SCIENCE 2011, VOL 20:131-139.
Chen Y, Sprung R, Tang Y, Ball H, Sangras B, Kim SC, Falck JR, Peng J, Gu W, Zhao Y (2007) Lysine propionylation and butyrylation are novel post-translational modifications in histones. Mol Cell Proteomics 6: 812-819.
Cheng Z, Tang Y, Chen Y, KimS, LiuH, Li SS,GuW, Zhao Y (2009) Molecular characterization of propionyllysines in non-histone proteins. Mol Cell Proteomics 8:45-52.
Garrity J, Gardner JG, Hawse W, Wolberger C, Escalante-Semerena JC (2007) N-lysine propionylation controls the activity of propionyl-CoA synthetase. J Biol Chem 282:30239-30245.
Starai VJ, Celic I, Cole RN, Boeke JD, Escalante-Semerena JC (2002) Sir2-dependent activation of acetyl-CoA synthetase by deacetylation of active lysine. Science 298:2390-2392.
Tanny JC, Moazed D (2001) Coupling of histone deacetylation to NAD breakdown by the yeast silencing protein Sir2: evidence for acetyl transfer from substrate to an NAD breakdown product. Proc Natl Acad Sci USA 98:415-420.
SABI
Switch mode to "source" to view source code for a mix of text and images.Images must be placed on the Amazon Server in the folder /biosyn35/images/userfiles/image/A brief history of firsts in nanotechnology: Nanotechnology started in 1959 when Feynman gave an after-dinner talk describing molecular machines built with atomic precision. In 1974, Taniguchi used the term "nano-technology" in a paper on ion-sputter machining. The molecular nanotechnology concept was coined by Drexler at MIT in 1977. The first technical paper on molecular engineering to manufacture with atomic precision was published in 1981, when the scanning tunneling microscope (STM) was invented. The Buckyball was discovered in 1985, and the first book on nanotechnology was published in 1986. During the same year, atomic force microscopy (AFM) was invented, and the first nanotechnology organization was formed. The first protein was engineered in 1987, and many firsts followed in nanotechnology, including the publication of the first textbook about the field in 1992, as well as the first nanomedicine book published in 1999. And it all starts with particles.
Proteins that make up the cells nanomachinery are just around 5 nanometers (nm) in size. These are the sizes of the smallest man-made nanoparticles. Their size allows them to be used as probes to study the cells’ biological processes. Typically, for biological applications, nanomaterials are selected for their optical and magnetic properties. However, nanomaterials are also applied for novel electronic, optoelectronis, and memory devices. Figure 1 illustrates the size of nanoparticles.
Some applications of nano-materials in biology and medicine are:Fluorescent biological labels for fluorescent signaling
Nanoparticles that usually form the core of nano-biomaterials can be composed of inorganic, polymeric materials or can be in the form of nano-vesicles surrounded by a membrane or a layer. The shapes of these particles can come in different morphologies. Furthermore, nanoparticles can be functionalized in multiple ways. Figures 3 and 4 show the graphical representation of different ways to functionalize nanoparticles. For example, as depicted in figure 3, the particles can be conjugated to different types of molecules, such as antibodies, green fluorescent protein (GFP), avidin, or streptavidin, as well as to DNA/RNA oligomers and gold particles or, as shown in Figure 4, contrast agents useful for CT/MRI imaging, radiotracers useful for PET/SPECT imaging, functionalized with drugs for targeted drug delivery or specific ligands, as well as special surfaces such as hydrophilic surfactants to enhance biocompatibility. It is thought that nanoparticles will play an increasing role in nanomedicine in the future. Nanomedicine applies nanotechnology with the goal to improve the quality of human lives. Useful medical applications of nanoparticles include improved drug delivery, such as protein, peptide, and oligonucleotide delivery in biological systems, nanoparticles to specific targets in tumors and cancers, and nanoparticles for tissue visualization to enhance surgical techniques or to visualize tumors. It is hoped that it will become possible in the near future to design nanorobots or nanomachines that allow for the repair of damage parts of the cell. More recently, many companies have begun to use nanotechnologies. The majority of the companies are small recent spinouts of various research institutions. Most of the companies are developing applications for the pharmaceutical industry, mainly to enhance or enable drug delivery. Other companies exploit quantum size effects in semiconductor nanocrystals to tag biomolecules or use gold nanoparticles for the labeling of various cellular parts. Cytodiagnostics, one such company, provides gold, silver, and magnetic nanoparticles with sizes ranging from 5 nm to 400 nm. These particles can be conjugated to biomolecules such as antibodies, BSA, KLH, and many others. Biosynthesis Inc. in Lewisville, Texas offers custom conjugation of antibodies or other proteins to this type of nanoparticles. To be able to perform custom conjugations, approximately 1-2 mg of purified and lyophilized antibody (or any other protein) is required. Services include: 1. antibody or protein sourcing, if needed; 2. conjugation of a customer protein to selected gold nanoparticles; and 3. Purification of the conjugate. Furthermore, oligonucleotides and other molecules can be conjugated to gold nanoparticles as well. Biosynthesis provides custom conjugation of single-stranded or double-stranded oligonucleotides to gold nanoparticles with sizes ranging from 5 nm to 200 nm. Final conjugate yield depends on both sizes of the oligo and nanoparticle. For a 20 mer oligo, the loading would be 0.2-1 OD/mg nanoparticle. The smaller the particle size, the higher yield. Services include: 1. synthesis of oligonucleotides for conjugation. The customers usually supplies the nucleotide sequence, and decides which terminal (5' or 3') will be attached to the gold surface, and Biosynthesis Inc. does the rest; 2. Conjugation of the oligonucleotide to a gold nanoparticle size of customer’s choice; 3. Purification of the conjugate. The following table shows a list of nanomedical technologies.
Cytodiagnostics, another company, offers a unique proprietary protocol that produces particles with uniform shapes and a narrow size distribution. The gold nanoparticle surface can be modified to allow for the conjugation to molecules such as biotin and other molecules of choice. Furthermore, particles can be functionalized with carboxyl, amine, and methyl groups, among others. ![]() Source: http://www.cytodiagnostics.com/gold_nanoparticle_products.php ![]() Silver Nanoparticles are also available with core sizes of 40 nm - 100nm Figure 6: Sizes of silver nanoparticles are illustrated.Source: http://www.cytodiagnostics.com/store/pc/Silver-Nanoparticles-c150.htm High-quality monodisperse silver nanoparticles with a narrow size distribution (CV <15%) are available as well. Nanosilver products are ideal for a wide array of applications such as “Conjugate Development,”, “Sensor Development,”, “Molecular Imaging’,’Surface Enhance Raman Spectroscopy (SERS),”, and“Bactericidal applications.” ![]() Source: http://www.cytodiagnostics.com/store/pc/Silver-Nanoparticles-c150.htm
The company Nanoprobes offers 1.4 nm Nanogold® particles. These are gold compounds that are not just adsorbed to proteins, like colloidal gold, but covalently reacts at specific sites under mild buffer conditions with molecules that are selected for conjugation. A well-defined product can be synthesized that can be purified chromatographically. Source: http://www.nanoprobes.com/products/LabRgts.html#feat
Robert A. Freitas Jr., What is nanomedicine? Nanomedicine: Nanotechnology, Biology, and Medicine 1 (2005) 2– 9 http://www.foresight.org/Nanomedicine/ |
Hybrid Peptides
Reference
Victor A. Gault, Vikas K. Bhat, Nigel Irwin1, and Peter R. Flatt; A Novel Glucagon-like Peptide-1 (GLP-1)/Glucagon Hybrid Peptide with Triple-acting Agonist Activity at Glucose-dependent Insulinotropic Polypeptide, GLP-1, and Glucagon Receptors and Therapeutic Potential in High Fat-fed Mice. THE JOURNAL OF BIOLOGICAL CHEMISTRY VOL. 288, NO. 49, pp. 35581-35591, December 6, 2013.
Native PAGE / Gel Electrophoresis Methods
Blue native PAGE or BN-PAGE
Blue native PAGE (BN-PAGE) is a native polyacrylamide gel electrophoresis technique in which the dye Coomassie Brilliant Blue provides the necessary charges to the protein complexes needed for electrophoretic separation so that they migrate into the gel employed. However, because Coomassie Blue binds to proteins it can act like a detergent which can cause protein complexes to dissociate <http://en.wikipedia.org/wiki/Dissociate>. In addition Coomassie Blue also potentially can quench the chemoluminescence or fluorescence of proteins containing prosthetic groups such as heme, chlorophyll or fluorescent dyes.
Clear native PAGE
Clear native PAGE (CN-PAGE) or Native PAGE allows separation of acidic water-soluble and membrane proteins using a polyacrylamide gradient gel. No charged dye is used here therefore the electrophoretic mobility of proteins in CN-PAGE is related to the intrinsic charge and shape of the proteins. The observed migration distance is depended on the protein charge, its size and the pore size of the gel. In general this method has a lower resolution than BN-PAGE but may offer advantages whenever the Coomassie dye will interfere with further analytical techniques. Furthermore, CN-PAGE is milder than BN-PAGE so it can retain labile supramolecular assemblies of membrane protein complexes that are dissociated under the conditions of BN-PAGE. However, to establish the best experimental conditions several trial runs maybe needed before the best separation conditions are found.
Quantitative preparative native continuous PAGE
Preparative native continuous gel electrophoresis (QPNC-PAGE) can be used to separate native, correctly folded protein complexes of interest. To be successful, the proper buffer system and apparatus will need to be selected or designed. For example, QPNC-PAGE can be used to identify and quantify metal cofactors comigrating with proteins in specific fractions. In addition, metalloproteins can be isolated and elucidated with the help of non-denaturing methods. Over the years many different gelelectrophoresis systems have been designed and are offer now by many commercial companies.
Locked Nucleic Acids (LNA) Vs. Bridged Nucleic Acids (BNA)
LNA (Locked Nucleic Acids)
BNA (Bridged Nucleic Acids)
2’-F-RNA.
Locked Nucleic Acids (LNA) gained its notoriety in being used in probes and siRNA. Currently Bio-Synthesis does not offer LNA monomers and they are now only available from Exiqon. A common question is whether there are other modified monomers that could offer the same properties as LNA or be better. One modified monomer that has gained notoriety is 2’-F-RNA. When 2’-F-RNA is incorporated into oligonucleotides, it increases the Tm of the duplex by about 1 to 2 oC per substitution. Just like LNA and most other sugar modified bases, 2’-F-RNA substituted oligos are more resistant to degradation both in vitro and in vivo. LNA received its name due to its ability to lock a nucleotide into the 3’-endo (North) conformation which is often found in the A-Form duplexes. However, while 2’-F RNA nucleotides also prefer a C3’- endo/north conformation unlike LNA 2’-F RNA nucleotides have the ability to undergo pseudorotation of the ribose.
A third and new modification that can be done to oligonucleotides is the incorporation of Bridged Nucleic Acids (BNA) monomers. Currently Bio-Synthesis exclusively offers custom oligonucleotides modified with BNA. BNA is closely related to LNA and developed by Takeshi Imanishi in Japan. 2’,4’-BNA modified oligonucleotides perform as well as and even better than LNA modified oligonucleotides. BNA has a very high target affinity, similar to or even higher than that of LNA and can increase Tm per modification by 5 to 6oC when binding to complementary RNA. Additionally BNA shows enhanced triplex formation, improved resistance to nuclease depredation, and more use in antisense and probe applications than LNA or 2’-F RNA.
Click Chemistry
Azides are useful electrophilic contributors in click chemistry reactions due to their ease of formation and stability. For this reason, alkyl azides undergo nearly no side reactions and are extremely stable in aqueous solution regardless of the presence of biological material. In addition, alkyne groups are also remarkably stable in biological mixtures, as long as they don’t have an activating group nearby, such as a carbonyl, which would make them susceptible to Michael-type addition reactions, especially with thiols.
CRISPR-Cas Systems
Type I and III CRISPR/Cas systems share specialized Cas endo-nucleases that process the pre-crRNAs. After maturation, each crRNA assembles into a large multi-Cas protein complex that recognizes and cleaves nucleic acids complementary to the crRNA.
The type II CRISPR Cas system processes pre-crRNAs using a different mechanism in which a trans-activating crRNA (tracrRNA) complementary to the repeat sequences in pre-crRNAs triggers processing of the double-stranded RNA-specific ribonuclease RNAse II in the presence of the Cas9 protein. The Cas9 protein appears to be the responsible part for crRNA-guided silencing of foreign DNA. The system works by incorporating short exogenous DNA sequences from the invading pathogen into specific loci of the host genome. At the time of transcription, these sequences are processed into crRNAs via pre-crRNAs. The crRNAs act as a guide for the Cas9 nuclease machinery. The Cas9 nuclease cleaves and inactivates the foreign DNA. The system requires two more pieces to function. A tracrRNA that forms base-pairs with the crRNA provides the substrate for the host's ribonuclease RNase III. This system can identify DNA sequences that are complementary to the crRNA and degrade them.
Glycosylated Peptide Synthesis
Methylene Blue Oligo Labeling
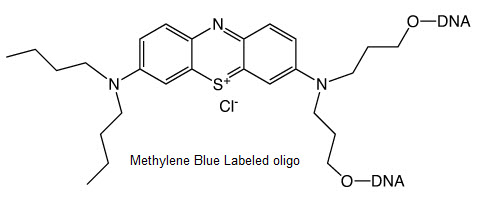
Methylene blue labeled oligo was utilized by several groups as redox reporter bound to DNA porbes in the detection of biological analytes.12-15
provided efficient electron transfer good sensitivity and stability in biosensor detection systems, at some situation showing better than ferrocene21.
The location of methylene blue (MB) in teh DNA probe seems to be critical for sensor performance. Internal labeled methylene blue probes have shown better signal suppression after target addition than end-labelled probes.13 Barton's group has shown the electrochemical resonse of methylene blue bound to dsDNA with a flexible linker and demonstrated both direct reduction at the electrode adn DNA-mediated reduction dependent on the n-stacking of methylene blue.22,23.
Intercalation properties of MB with DNA have been widely investigated but only with free MB molecules in solution.24-28 DNA structures with an intercalated MB still have to be considered, and novel binding chemistries have to be explored. However, only a post-synthesis functionalization of DNA with an NHS-ester derivative has been used to incorporate MB into oligonucleotides.22,29,30 This method is limited by the requirement for amino functions on the oligonucleotide chain. Although the introduction of amino groups is relatively straightforward with the appropriate phosphoramidites, the procedure requires an additional deprotection step followed by an additional coupling/purification procedure to incorporate the MB. This final step could be tedious due to the trend of MB to interact with DNA via electrostatic and π-stacking interactions with the phosphate backbone and nucleic bases (in particular with guanine residues), respectively, thus decreasing the efficiency of covalent incorporation.31,32
Bio-Synthesis provide methylene blue oligo labeling by covalently introduce into the any position of an oligonucletoide sequence.
References:
- L. Anderson, S. M. Wittkopp, C. J. Painter, J. J. Liegel, R. Schreiner, J. A. Bell and B. Z. Shakhashiri, Journal of Chemical Education, 2012, 89, 1425-1431.
- M. Wainwright, Dyes and Pigments, 2007, 73, 7-12.
- M. Wainwright and R. M. Giddens, Dyes and Pigments, 2003, 57, 245-257.
- 4. M. Wainwright, K. Meegan, C. Loughran and R. M. Giddens, Dyes and Pigments, 2009, 82, 387-391.
- D. E. Wetzler, D. Garcia-Fresnadillo and G. Orellana, Physical Chemistry Chemical Physics, 2006, 8, 2249-2256.
- E. M. Tuite and J. M. Kelly, Journal of Photochemistry and Photobiology B: Biology, 1993, 21, 103-124.
- J.-L. Ravanat, P. Di Mascio, G. R. Martinez, M. H. G. Medeiros and J. Cadet, Journal of Biological Chemistry, 2000, 275, 40601-40604.
- K. J. Mellish, R. D. Cox, D. I. Vernon, J. Griffiths and S. B. Brown, Photochemistry and Photobiology, 2002, 75, 392-397.
- J. E. Harris and A. Peters, Quarterly Journal of Microscopical Science, 1953, s3-94, 113-124.
- J. I. Clifton and J. B. Leikin, American Journal of Therapeutics, 2003, 10, 289-291.
- M. Oz, D. E. Lorke and G. A. Petroianu, Biochemical Pharmacology, 2009, 78, 927-932.
- J. Wang, F. Wang and S. Dong, Journal of Electroanalytical Chemistry, 2009, 626, 1-5.
- A. A. Lubin, B. Vander Stoep Hunt, R. J. White and K. W. Plaxco, Analytical Chemistry, 2009, 81, 2150-2158.
- L. Zhu, R. Zhao, K. Wang, H. Xiang, Z. Shang and W. Sun, Sensors, 2008, 8, 5649-5660.
- W. Yang and R. Y. Lai, Langmuir, 2011, 27, 14669-14677.
- A. A. Rowe, K. N. Chuh, A. A. Lubin, E. A. Miller, B. Cook, D. Hollis and K. W. Plaxco, Anal Chem, 2011, 83, 9462-9466.
- B. R. Baker, R. Y. Lai, M. S. Wood, E. H. Doctor, A. J. Heeger and K. W. Plaxco, Journal of the American Chemical Society, 2006, 128, 3138-3139.
- F. Ricci, R. Y. Lai and K. W. Plaxco, Chemical Communications, 2007, 3768-3770.
- K. Hsieh, R. J. White, B. S. Ferguson, K. W. Plaxco, Y. Xiao and H. T. Soh, Angewandte Chemie International Edition, 2011, 50, 11176-11180.
- R. J. White, H. M. Kallewaard, W. Hsieh, A. S. Patterson, J. B. Kasehagen, K. J. Cash, T. Uzawa, H. T. Soh and K. W. Plaxco, Analytical Chemistry, 2011, 84, 1098-1103.
- D. Kang, X. Zuo, R. Yang, F. Xia, K. W. Plaxco and R. J. White, Analytical Chemistry, 2009, 81, 9109-9113.
- C. G. Pheeney and J. K. Barton, Langmuir, 2012, 28, 7063-7070.
- C. G. Pheeney and J. K. Barton, J. Amer. Chem. Soc., 2013, 135, 14944-14947.
- S. O. Kelley, J. K. Barton, N. M. Jackson and M. G. Hill, Bioconjugate Chemistry, 1997, 8, 31-37.
- A. Erdem, K. Kerman, B. Meric and M. Ozsoz, Electroanalysis, 2001, 13, 219-223.
- D. Ozkan, A. Erdem, P. Kara, K. Kerman, J. Justin Gooding, P. E. Nielsen and M. Ozsoz, Electrochemistry Communications, 2002, 4, 796-802.
- J. Gu, X. Lu and H. Ju, Electroanalysis, 2002, 14, 949-954.
- E. L. S. Wong, P. Erohkin and J. J. Gooding, Electrochemistry Communications, 2004, 6, 648-654.
- A. Abi and E. E. Ferapontova, Journal of the American Chemical Society, 2012, 134, 14499-14507.
- J. Jähnchen, M. G. M. Purwanto and K. Weisz, Biopolymers, 2005, 79, 335-343.
- M. Ortiz, A. Fragoso, P. J. Ortiz and C. K. O'Sullivan, Journal of Photochemistry and Photobiology A: Chemistry, 2011, 218, 26-32.
- M. Hossain, A. Kabir and G. Suresh Kumar, Dyes and Pigments, 2012, 92, 1376-1383.
- G. Chatelain, C. Chaix, H. Brisset, C. Moustrou, F. Fages and B. Mandrand, Sensors and Actuators B: Chemical, 2008, 132, 439-442.
- G. Chatelain, M. Ripert, C. Farre, S. Ansanay-Alex and C. Chaix, Electrochimica Acta, 2012, 59, 57-63.
- C. Chaix and G. De Crozals: February 2013, French patent, University Lyon1/CNRS, PCT/FR2013/050356.
Fluorescent Base Analog for DNA or RNA Synthesis
Pyrrollo-dC and Pyrrollo C bases, these fluroescent analogues code efficiently as C, it is an ideal probe of DNA structure and dynamics.
- It base-pairs as a normal dC nucleotide. An oligo fully substituted with pyrrolo-dC has the same Tm as the control dC oligo with the same specificity for dG
- Its small size does not perturb the structure of the DNA helix and it is well tolerated by a number of DNA and RNA polymerases.
- It is highly fluorescent and its excitation and emission are wellto the red of most fluorescent nucleotide analogs, which eliminates or reduces background fluorescence from proteins.
The spectrral properties of pyrrollo-dC, coupled with its unique base-pairing ability, making this fluorescent analog extremely valuable in probing DNA structure. When the pyrrolo-dC is base-paired, its fluorescence is significantly quenched through waht is most likely base stacking or dG interaction.
QY | λ | ε(L/mol.cm) | |
Single-stranded | 0.07 | 260 nm | 4000 |
347 nm | 3700 | ||
double-stranded | 0.02 |
The quenching of pyrrolo-dC allows local structural changes to be probed with great sensitivity. Using pyrrolo-dC, Liu and Martin8 have characterized the transcription bubble in elongation complexes of T7 RNA Polymerase to single-base resolution by observing roughly a two-fold increase in fluorescence as the polymerase induces melting. By starving the T7 RNA Polymerase of specific nucleoside triphosphates, the enzyme could be stalled at specific sites, producing 'fluorescence snapshots' of the complex, and yielding detailed information on the nature of the transcription bubble and heteroduplex.
Work is still progressing in evaluating the effect of this modified fluorescent nucleoside in biological systems and will be reported. However, a few comments on our findings to date may be of interest. Oligonucleotides containing pyrrolo-dC act as efficient primers and the PCR products appear to be identical for primers with 0 to 5 pyrrolo-dC residues replacing dC. Preliminary data indicate that pyrrolo-dC codes as dC in PCR experiments. And very preliminary evidence indicates that pyrrolo-dC triphosphate is incorporated efficiently by Taq polymerase and is incorporated specifically opposite dG.
References- S.C. Srivastava, S.K. Raza, and R. Misra, Nucleic Acids Res, 1994, 22, 1296-304.
- M.E. Hawkins, W. Pfleiderer, O. Jungmann, and F.M. Balis, Anal Biochem, 2001, 298, 231-240.
- M.E. Hawkins, W. Pfleiderer, F.M. Balis, D. Porter, and J.R. Knutson, Anal Biochem, 1997, 244, 86-95.
- S.L. Driscoll, M.E. Hawkins, F.M. Balis, W. Pfleiderer, and W.R. Laws, Biophys J, 1997, 73, 3277-86.
- R. Charubala, et al., Nucleos Nucleot, 1997, 16, 1369-1378.
- J.M. Jean and K.B. Hall, Proc Natl Acad Sci U S A, 2001, 98, 37-41.
- J. Woo, R.B. Meyer, Jr., and H.B. Gamper, Nucleic Acids Res, 1996, 24, 2470-5.
- C. Liu and C.T. Martin, J Mol Biol, 2001, 308, 465-75.
- C. Liu and C.T. Martin, J Biol Chem, 2002, 277, 2725-31.
Amino Acid Analysis to elucidate Tryptophan Pathways
By Klaus D. Linse
The Serotonin Pathway
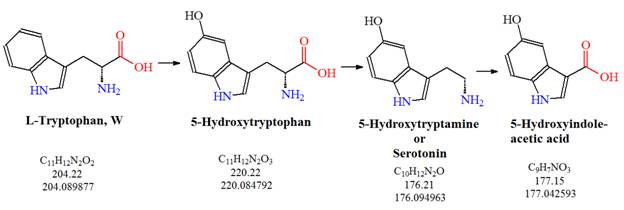
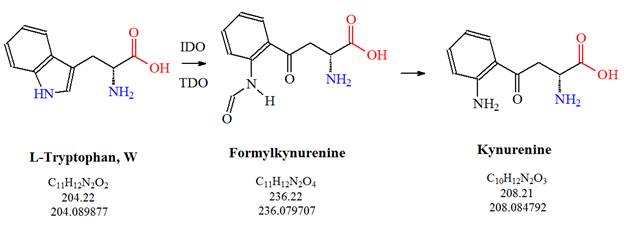
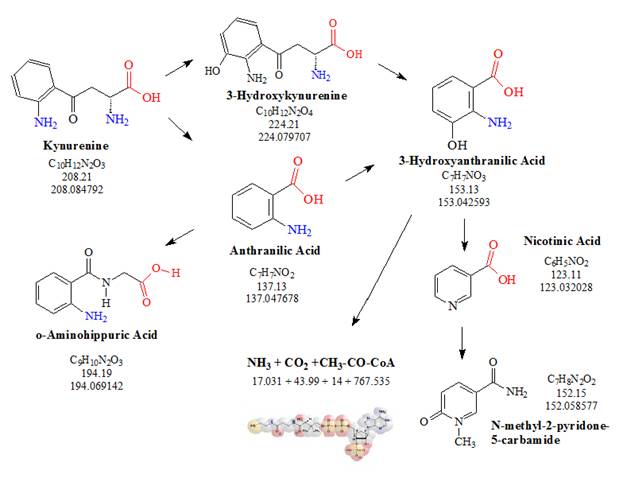
Please visit our website for more information on Amino Acid Analysis
Geier EG, Schlessinger A, Fan H, Gable JE, Irwin JJ, Sali A, Giacomini KM.; Structure-based ligand discovery for the Large-neutral Amino Acid Transporter 1, LAT-1. Proc Natl Acad Sci U S A.<http://www.ncbi.nlm.nih.gov/pubmed/23509259> 2013 Apr 2;110(14):5480-5. doi: 10.1073/pnas.1218165110. Epub 2013 Mar 18.
Hayashi K, Jutabha P, Endou H, Sagara H, Anzai N.; LAT1 is a critical transporter of essential amino acids for immune reactions in activated human T cells. J Immunol.<http://www.ncbi.nlm.nih.gov/pubmed/24038088> 2013 Oct 15;191(8):4080-5. doi: 10.4049/jimmunol.1300923. Epub 2013 Sep 13.
Rishabh Kapila, Kikeri Seetaramaih Nagesh, Asha Iyengar, Divyalakshmi Diabetes and Oral Changes: The Tryptophan Metabolism Link? Journal of Clinical and Diagnostic Research. 2012 May (Suppl-1), Vol-6(3):517-520.
Walter H. Kaye, Ursula F. Bailer, Guido K. Frank, Angela Wagner, Shannan E. Henry; Brain imaging of serotonin after recovery from anorexia and bulimia nervosa. Physiology & Behavior 86 (2005) 15 - 17.
ALFRED F. MICHAEL, KEITH N. DRUMMOND, DORIS DOEDEN, JOHN A.
ANDERSON, AND ROBERT A. GOOD; Tryptophan Metabolism in Man. Journal of Clinical Investigation Vol. 43, No. 9, 1964.
Web links
The "Tryptophan metabolism - Reference pathway" - http://www.genome.jp/kegg-bin/show_pathway?map00380+C00024
Tryptophan Metabolism - http://pathman.smpdb.ca/pathways/SMP00063/pathway
MetaCyc, a database of nonredundant, experimentally elucidated metabolic pathways. - http://metacyc.org/
Dolastatin Peptides
Reference
Hendrik Luesch, Richard E. Moore, Valerie J. Paul, Susan L. Mooberry, and Thomas H. Corbett; Isolation of Dolastatin 10 from the Marine Cyanobacterium Symploca Species VP642 and Total Stereochemistry and Biological Evaluation of Its Analogue Symplostatin 1. J. Nat. Prod., 2001, 64 (7), pp 907-910. DOI: 10.1021/np010049y.
David J. Newman and Gordon M. Cragg; Marine Natural Products and Related Compounds in Clinical and Advanced Preclinical Trials. J. Nat. Prod. 2004, 67, 1216-1238.
David J. Newman and Gordon M. Cragg; Marine-Sourced Anti-Cancer and Cancer Pain Control Agents in Clinical and Late Preclinical Development. Mar. Drugs 2014, 12(1), 255-278; doi:10.3390/md12010255 Review.
Therapeutic Peptides for Treating Cancer
The three therapeutic peptides goserelin, leuprolide and octreotide are already used to treat cancer, either directly or in the treatment of episodes associated with cancer tumors.
Goserelin acetate, the salt of a decapeptide, is an injectable gonadotropin releasing hormone superagonist, also known as a luteinizing hormone releasing hormone agonist. A superagonist is a compound that can bind to a receptor and activate it more strongly than the naturally produced activating molecule. Goserelin is used to suppress production of the sex hormones in the treatment of breast and prostate cancer and to treat hormone-sensitive cancers of the breast and prostate.
Leuprolide acetate is a gonadotropin-releasing hormone or luteinizing-hormone-releasing hormone analog used in the treatment of hormone-responsive cancers such as prostate cancer or breast cancer, estrogen-dependent conditions, precocious puberty, and to control ovarian stimulation in In-Vitro Fertilization.
Octreotide, a octapeptide, is a somatostatin mimic. The peptide hormone somatostatin regulates the endocrine system, affects neurotransmission and cell proliferation via interaction with G protein-coupled somatostatin receptors and inhibits the release of numerous secondary hormones. Octreotide is also a more potent inhibitor of growth hormone, glucagon, and insulin than the natural hormone. The salt form of the peptide, octreotide acetate, is approved by the Food and Drug Administration (FDA) to be used as an injectable depot formulation for the treatment of growth hormone producing tumors, pituitary tumors that secrete thyroid stimulating hormone, diarrhea and flushing episodes associated with carcinoid syndrome, and diarrhea in patients with vasoactive intestinal peptide-secreting tumors.
In addition, host defense peptides are known to be present in most living species. These peptides exhibit a diverse range of functions including the direct killing of pathogens, immune-modulating properties and some host defense peptides have been reported to be potent against cancer cells and animal models have shown that several host defense peptides can confer complete and permanent tumor regression of different types of solid tumors.
Peptides that inhibit or modulate cell signaling interactions needed for cancer growth may also be used as anticancer agents. These types of therapeutic peptides have great potential as anti-cancer agents because they can be rationally designed to increase their target specificity and the following types have been reported so far: receptor-interacting compounds, inhibitors of protein-protein interaction, enzymes inhibitors; nucleic acid-interacting compounds, as well as active peptides for which no mechanism of action has been found yet.
Natural bioactive peptides derived from different foods represent another source of health-enhancing or cancer fighting compounds. Different studies indicate that it is possible that many of these peptides may be released during digestion in the human stomach or during food processing from various plant and animal proteins, especially milk, soy, and fish proteins. In addition, a number of studies focusing on marine bioactive peptides have identified many peptides and depsipeptides with anticancer potential. These types of peptides been extracted from various marine animals like tunicates, sponges, soft corals, sea hares, nudibranchs, bryozoans, sea slugs, and other marine organisms.
Drug design approaches that capitalize on structure-activity relationships found in natural inhibitory peptides are powerful tools for the discovery of novel, stable peptide mimics that exhibits unique high-affinity binding to human protein or peptide receptor targets for the development of future anticancer peptide therapeutics.
Peptides used as therapeutic agents for the treatment of cancer have gained momentum in recent years. The activity of different peptides that can be used for this type of treatment is attributed to mechanisms that restrict tumor growth. These can include the inhibition of angiogenesis, protein-protein interactions, enzymes, proteins, signal transduction pathways, or gene expression, or anticancer peptides that act as specific receptor antagonist. In addition, some peptides may also mediate the induction of apoptosis, the programmed cell death, in tumors.
References:
Albericio & Kruger;Therapeutic peptides. Future Med. Chem. (2012) 4(12), 1527-1531.
Diana Gaspar, A. Salomé Veiga and Miguel A. R. B. Castanho < http://www.
Front. Microbiol., 01 October 2013 | doi: 10.3389/fmicb.2013.00294.
Thundimadathil J.; Cancer treatment using peptides: current therapies and future prospects. J Amino Acids. < http://www.ncbi.nlm.
Guadalupe-Miroslava Suarez-Jimenez, Armando Burgos-Hernandez and Josafat-Marina Ezquerra-Brauer; Bioactive Peptides and Depsipeptides with Anticancer Potential: Sources from Marine Animals. Mar. Drugs 2012, 10, 963-986; doi:10.3390/md10050963.
Anticancer Peptides
References
Diana Gaspar, A. Salomé Veiga and Miguel A. R. B. Castanho<http://www.
Thundimadathil J.; Cancer treatment using peptides: current therapies and future prospects. J Amino Acids.<http://www.ncbi.nlm.